C* - An unknown star
- Andreas
- Oct 15, 2018
- 5 min read
Updated: Apr 27, 2020
C* (pronounced „C-Star“), is one of the dominant digital flight control laws for Fly-By-Wire (FBW) transport aircraft. It has its roots in the 1960’s in the United States [1] [4] and is used by several of the most important aircraft manufacturers for pitch control. A large European aircraft manufacturer uses a version closely related to the original C* idea, while a large U.S. manufacturer uses a more modified version to obtain a rather conventional aircraft behaviour and calls it C*U [1].
That should be enough motivation for us to shed some light on the underlying magic. Now if you are not into engineering, no worries, there will only be two equations in this article, I promise!
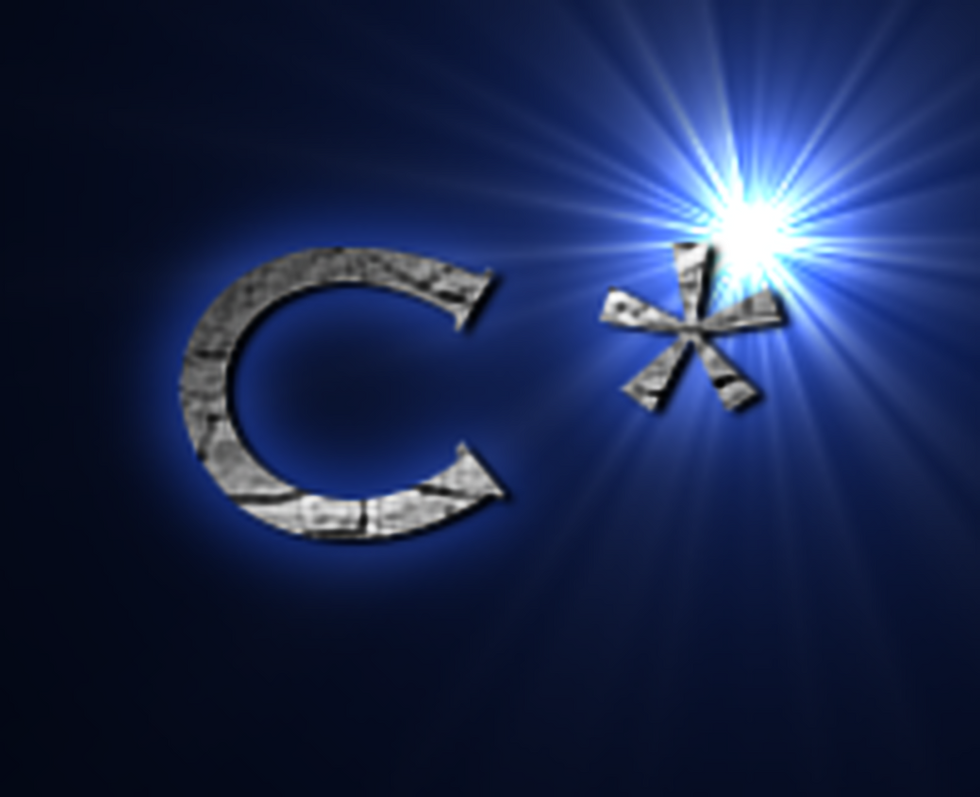
Figure 1: C*, a magic concept
FBW technology is in fact not a recent development at all, as the general definition refers to electrical signalling of control surface actuators [1]. Such an aircraft would still behave relatively conventionally. The probably earliest application of electrical signalling to flight control servos was achieved by Tupolev on the ANT-20 aircraft during the 1930’s (!) [5]. Later examples were the Avro Arrow CF-105 in the 1950’s, or the NASA F-8 program, the latter actually introduced the digital FBW technology [2].
Electrical signalling really forms the foundation of every aerospace engineer’s dream: Being able to change what the pilot controls ;-) The introduction of digital FBW systems has provided the freedom for an infinite amount of possible control laws.
The C* control idea
A pilot primarily senses changes in load factor and attitude [1] [3] [4]. Now the flight dynamics dictate that at “high” airspeeds, the load factor change is more noticeable, whereas at “low” speeds, the attitude change dominates [3] [4]. And this is already the key idea of the C* control law: To create a parameter (C*), that represents a linear combination of load factor and pitch-rate [4]. This makes it relatively easy to maintain a stable flight path in cruise, while maintaining an intuitive behavior for maneuvering. The problem of how to “trade” pitch-rate priority for load factor priority was originally solved by introducing a “crossover speed”. At that speed, the load factor demand and the pitch-rate demand are weighted equally [1] [4].

Figure 2: C* control architecture (simplified), based on [1] [4]
Figure 2 depicts a simplified version of the C* concept. The pilot controls a parameter, called C*, that is composed of the normal load factor (green) and the normal load factor that would be felt, if the aircraft was travelling at the crossover speed Uco and subject to the current pitch rate (blue) (wings level). The ingenuity here: If the aircraft travels at Uco, then the two parts are equally weighted, if the aircraft is faster than Uco, the normal load factor demand dominates and if the aircraft is slower than Uco, the pitch-rate demand dominates [1][4]. The C* error is then fed through an integrator before being transmitted to the elevator. This mainly serves the purpose of reducing steady-state error. (If there is a small persistent error, the elevator deflection will steadily increase). Gains are not shown for simplicity.
For the mathematically inclined, it is obvious that this control law does not possess any “speed-stability” and thus fails to comply with basic airworthiness requirements [1]. A reduction of thrust will result in an increasing angle of attack without altitude loss. Looking at the aerodynamics, it is quite apparent that this will go on for a while, before coming to an abrupt end. Such a deficiency is usually accounted for by introduction of some sort of “envelope protection”, which ultimately restores a safe aircraft behaviour. In such a control law, a conventional trimming by the pilot makes no longer sense and automatic trimming is usually introduced [1][4].
A side note: A conventional aircraft is stable in angle of attack, not airspeed [1] [6], but if the load factor is kept constant, it seems that there is a “trim speed” (more on that in another article…)
The C*U control modification
Not all manufacturers and pilots like the pure C* control and so a very popular modification includes the re-introduction of “speed stability”. The “U” refers to the flight dynamics terminology for the velocity in the forward direction [1] [4]. It is important to realize that this is still a digital flight control law, based on the C* concept, but it includes a mathematical term to create a conventional aircraft behavior, while maintaining the good handling qualities of the C* control law [1]. A new term “Uerr” is added, which is nothing else that the difference between the “trim speed” and the current CAS. This creates a pitching moment similar to a conventional aircraft, if the CAS deviates from the “trim speed” [1].

Figure 3: C*U control architecture (simplified), based on [1]
Figure 3 above shows a C*U concept with the introduction of a “trim speed”. In the practical implementation of a particular U.S. manufacturer, the trim switches on the yoke do not cause the stabilizer to move directly, but just change the value of the “trim speed” [1]. For a pilot, the aircraft behaves very similar to a conventional aircraft.
A step further
When re-examining the idea of making it easy to maintain a certain flight path, the original C* algorithm is not quite perfect, since an external disturbance that results in a flight path deviation is not automatically compensated [1]. Some aircraft manufacturers have developed a so-called Flight Path Angle Rate Command (FPARC) control law that also takes into account such deviations and thus makes pilot life even easier [1]. Such an aircraft simply maintains the current flight path vector without pilot input. This control law in particular shows that a pilot is more and more pushed into the role of a strategic decision maker and less involved in the immediate control of aerodynamic control surfaces…
The control laws described above are all ingenious in their own way and I will leave it to the reader to decide which one fits best. The future will show what other developments we might be faced with and I think it is important for pilots to have a conceptual understanding of what they are actually controlling. Additionally, we should never forget that no matter how sophisticated a flight control law might be, there is no flight control law that would enable us to overcome basic physical limitations...
This short article is by far not all-encompassing, but I hope it provided some insights into the world of electrons between the yoke and the elevator.
Rev/20181015
References
[1] D. Niedermeier, A. Lambregts, 2009, Fly-by-Wire augmented manual control – basic design considerations, 28th International congress of the aeronautical sciences
[2] J. E. Tomayko, 2000, Computers take flight: A history of NASA’s pioneering fly-by-wire project, The NASA history series
[3] E. Field, 1993, The application of a C* flight control law to large civil aircraft, College of aeronautics, Cranfield
[4] J. P. Sutherland, 1968, fly-by-wire flight control systems, Air Force flight dynamics laboratory, Ohio
[5] http://www.tupolev.ru/en/aircrafts/ant-20_(maxim_gorky) (10.10.2018)
[6] J. Russel (1996), Performance & Stability of aircraft, 1st edition, Butterworth-Heinemann