How close can the mountains really be? Every pilot operating in mountainous terrain will sooner or later be confronted with this question. Even more so, as the number of Performance Based Navigation (PBN) procedures is steadily growing. The topics discussed here apply to PBN in general and are also relevant outside of the hilly terrain. The main goal of this article is to make to technical content of PANS-OPS and the ICAO PBN manual more accessible.
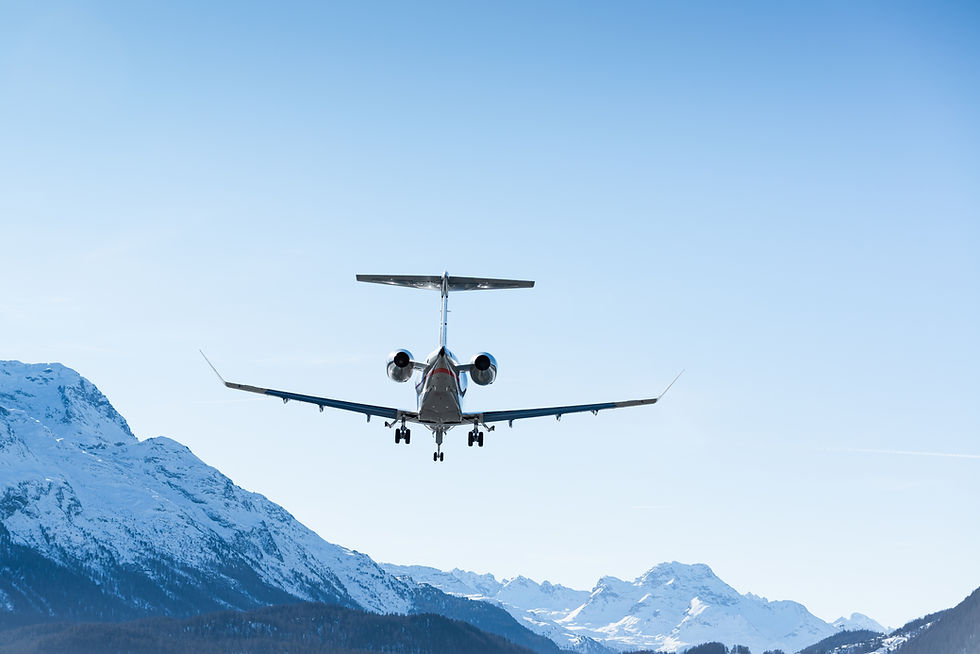
The Performance Based Navigation (PBN) concept has been well-established for years now and most pilots find it straightforward to fly such procedures. And yet, it can be very hard to find specific information about the error budgets and obstacle clearances involved. Why is that? Well, one aspect certainly is the fact that the information is distributed across a plethora of documents, and it can be quite challenging for flight crews to keep up with the ever-evolving regulatory guidance.
The PBN error budget – lateral
Before we dive into details, let us remind ourselves about the different lateral error components:
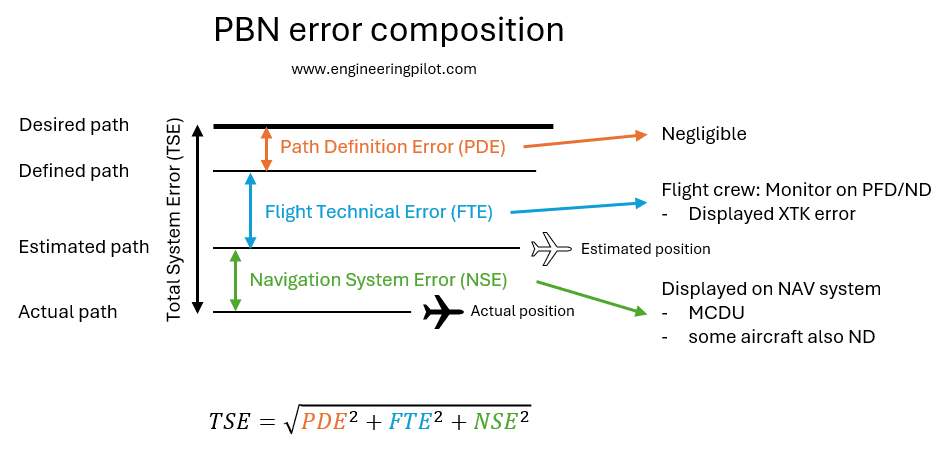
As seen above, the lateral Total System Error (TSE) consists of several sub-errors. It is important to realize that the dominant errors are the Flight Technical Error (FTE) and the Navigation System Error (NSE), both represent statistical quantities that are approximated using a Gaussian distribution [2]. They cannot simply be added algebraically, a special procedure using Root Sum Squared (RSS) must be applied.
In very simple terms, the TSE components can be described as follows:
The Path Definition Error (PDE)
Difference between the desired path and the defined path. Typical contributions are:
- Arinc 424 leg types (VA, Fly-by etc.)
- Magnetic variation mismatch
- Geodetic datum differences
The PDE can be ignored for all practical purposes, as it is very small.
The Flight Technical Error (FTE)
Difference between the defined path and the estimated path. Typical contributions are:
- Flight crew / autopilot precision
- Display errors
The FTE is usually shown on the Primary Flight Display (PFD) and/or Navigation Display (ND) depending on the installation.
The Navigation System Error (NSE)
Difference between the estimated path and the actual path. Typical contributions are:
- Navigation infrastructure
- Navigation system accuracy
The NSE is typically shown on the navigation system screen (MCDU), although some aircraft also depict it on the ND.

For the flight crew, it is important to understand their main task: Control and monitor the FTE. Certain assumptions have been made during the procedure design.
What are the design-assumptions for the FTE? PANS-OPS vol. 2 provides the answer [2]:
FTE95 design values:
RNAV: ½ RNAV value
RNP>=0.5: ½ RNP value
RNP<=0.5: 0.25 NM
For the keen observer, two things are immediately apparent:
If the TSE is within the RNAV/RNP value for 95% of the time, what about the remaining 5%? Obviously there must be some form of margin...
If the FTE has a design value, the flight crew must be provided with guidance on how to monitor the FTE
Both points are elaborated below...

On-board performance monitoring and alerting – but how?
As pilots we are aware that RNP procedures require On-Board Performance Monitoring and Alerting (OBPMA) while RNAV procedures do not [1]. But what exactly does it mean? It turns out, implementations can vary! The following table provides an overview of the monitoring and alerting requirements:
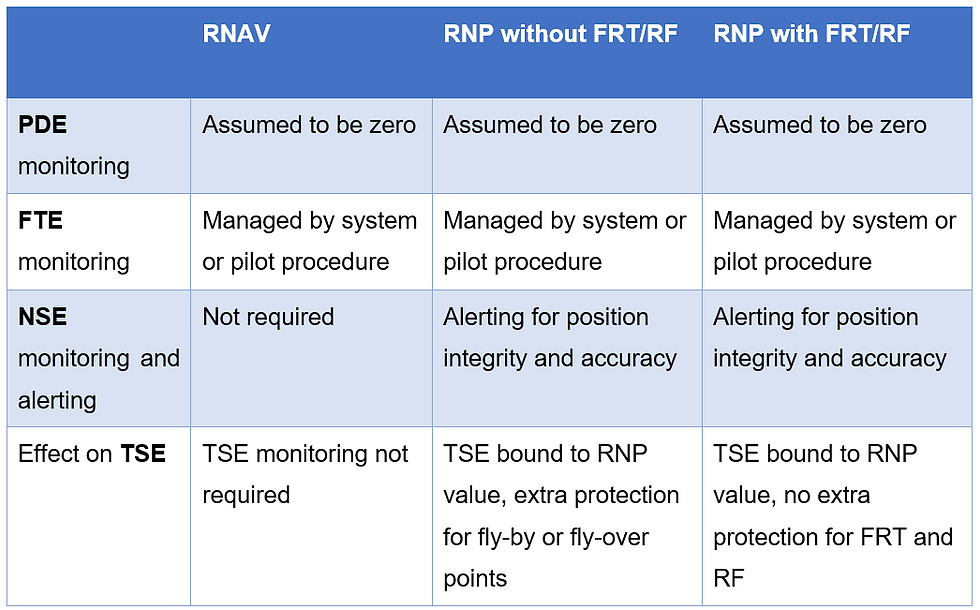
The ICAO PBN manual dictates [1]:
“The RNP system must provide a means to support TSE monitoring. The system must provide an alert to the flight crew when the accuracy requirement is not met or when the probability that the TSE exceeds two times the RNP value (such as 2 × RNP) is greater than one in 100,000 (that is, 1×10–5).”
And crucially:
“Some aircraft require the flight crew to monitor FTE. This requirement supports the monitoring of TSE performance when the aircraft offers an alert on the 95 per cent estimated lateral navigation accuracy, ensuring that the risk of the overall TSE (including the monitored NSE) exceeding 2 RNP will remain below one in 100,000 (that is, 1×10-5).”

The statistics – where do the 95% come from?
At some point during PBN training, most pilots will have come across the 95%-interval and very often, this number seems to “stick” to our heads. But what is this all about? The errors involved in these procedures are approximated using a gaussian distribution, which describes a random variable with a specific variation around a mean value [3]. How large the “variation” is can be described using a parameter called “standard deviation” or sigma σ. It can be shown that one-sigma deviation around the mean will contain 68.2% of all values. Similarly, a two-sigma deviation will contain 95.4% of all values, hence the 95% [3].
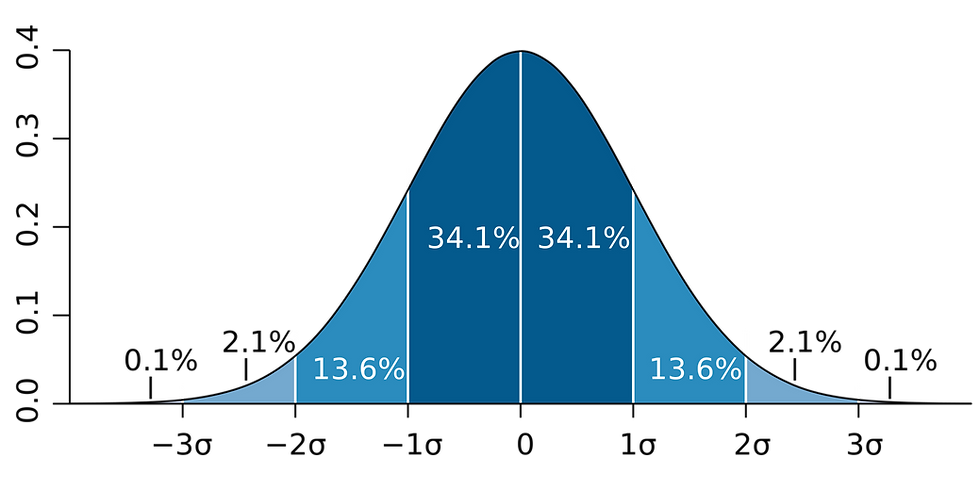
Several points are important:
This distribution is only an approximation of reality used for procedure design and is not based on a large-scale data set [2]
When adding multiple random variables variables, a special procedure is required (RSS)
Additional buffer values must be added to cover “outliers”.
The obstacle clearance
The method applied by PANS-OPS to determine the obstacle clearance area for PBN procedures is somewhat a mixture of legacy concepts and PBN-specific modifications. Fundamentally, the RNP/RNAV-value determines a parameter called Cross-Track Tolerance (XTT). This is then modified to include a 3-sigma interval and a buffer is added to cover outliers [2]. This results in the area semi-width (1/2 A/W). The factor of 1.5 is no coincidence: It is the extension of the two-sigma interval to include 3-sigma. The method is summarized in Figure 4.
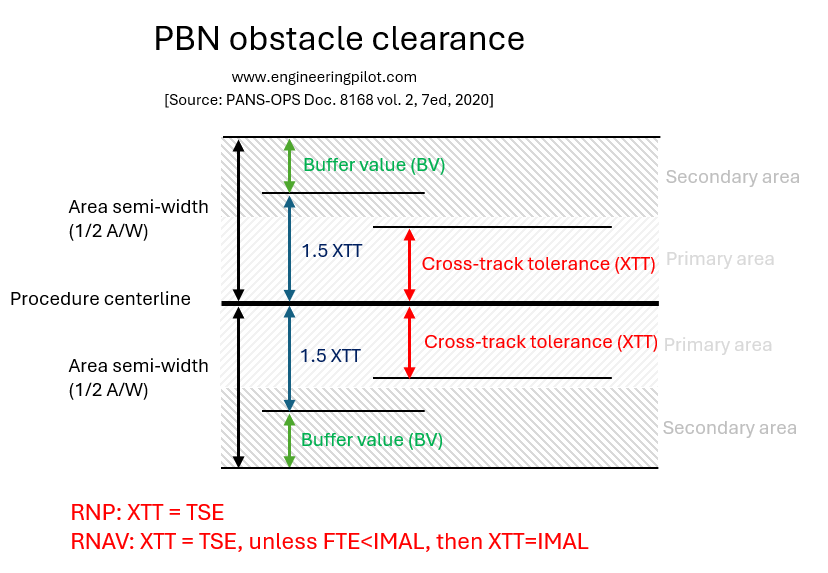
For RNP procedures the design is straight-forward: XTT is based on TSE.
For RNAV procedures the XTT is usually based on TSE. A special case applies, if GNSS is used to achieve RNAV capability: If the FTE becomes smaller than the Alarm Limit (IMAL) of the GNSS receiver, the XTT is based on the IMAL [2]. This leads to the somewhat “odd” numbers for RNAV 5 in the tables below.
The concept of primary and secondary area is also applied in PBN, albeit with some restrictions. Both (primary and secondary) are referenced to the area semi-width. The tables below provide a summary of the buffer values and of the resulting area semi-width (1/2 A/W):
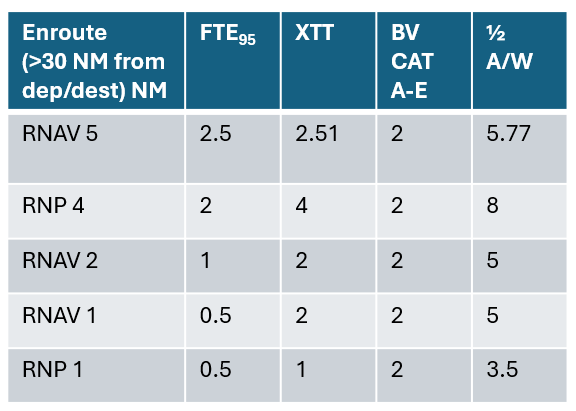
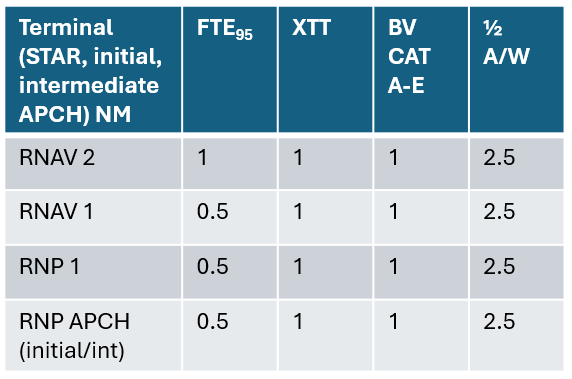

The tables are by no means complete but they may provide a good idea of the order of magnitude of the parameters involved. For helicopter (cat H) tolerances refer to [2].
Is there a “vertical RNP”?
Oddly enough, there is no PBN regime in the vertical domain [1]. Dautermann [4] investigated the possibility of extending the RNP philosophy to the vertical domain and the interested reader is referred to [4]. This does not imply that there are no errors. To the contrary, the vertical domain is crucial, especially during approach. Figure 5 below depicts an overview of the errors that are present in the vertical domain during an approach scenario. Note the similarity to the lateral domain and the fact that the horizontal NSE has a coupling effect into the vertical domain.

The Altimetry System Error (ASE) is addressed through certification and maintenance requirements as it is invisible to the flight crew. The flight crew’s main task here is to monitor the vertical FTE. Similar to the horizontal domain, the vPDE is negligible. The procedure design accounts for the Horizontal Coupling Error (HCE).
Vertical FTE tolerances
In Europe, the rules are as follows: The aircraft should not descend more than 75 ft below the baro-VNAV profile or half-scale deflection, where angular deviation is used. Furthermore, the aircraft should not be more than 75 ft above the baro-VNAV profile or half-scale deflection, where angular deviation is used, during the last 1000 ft above the aerodrome [5].
Adjustment of horizontal and vertical deviation scales
Depending on the kind of approach operation, the deviation scales are adjusted to limit the distribution of the FTE. This is sometimes referred to as “angular” or “linear” operation.

From a pilot’s perspective, the adjustment of deviation scales is a crucial element of an approach procedure, as it can usually be easily perceived and has to be checked for correctness by the flight crew. A more in-depth analysis of the obstacle clearance requirements can improve the situational awareness, especially regarding the elements that are not readily apparent during flight operation.
References
[1] | ICAO, «PBN Manual doc 9613 5th ed,» 2023. |
[2] | ICAO, «PANS-OPS doc. 8168, vol. 2 Construction of Visual and Instrument Flight Procedures,» 7th ed. 2020. |
[3] | Wikipedia, «Normalverteilung,» 2024. [Online]. Available: https://de.wikipedia.org/wiki/Normalverteilung#/media/Datei:Standard_deviation_diagram.svg. [Zugriff am 2024]. |
[4] | T. Dautermann, «Extending Required Navigation Performance to Include Time Based Operations and the Vertical Dimension,» Journal of The Institute of Navigation, Bd. 63, Nr. 1, 2016. |
[5] | EU commission, «Commission regulation 965 (EU-OPS),» 2023. |
[6] | ICAO, «Nav specifications,» Presentation (public domain), 2015. |