One of the most undervalued aircraft parts is hidden in plain sight: The cover that houses the sensitive antennas in front of the flight deck. It is referred to as the radome, a portmanteau of radar and dome. It will get everyone’s attention, once it stops doing its job. Enough reason for us to have a closer look.

Design aspects
The aircraft manufacturer has a multitude of problems to solve here:
Protect the antennas from environmental hazards
Minimize RF distortions
Minimize the aerodynamic drag
Dissipate static and direct lightning
It takes a special piece of equipment to achieve that. There are compromises between the different goals, as the ideal solution for one aspect might be unacceptable for another.
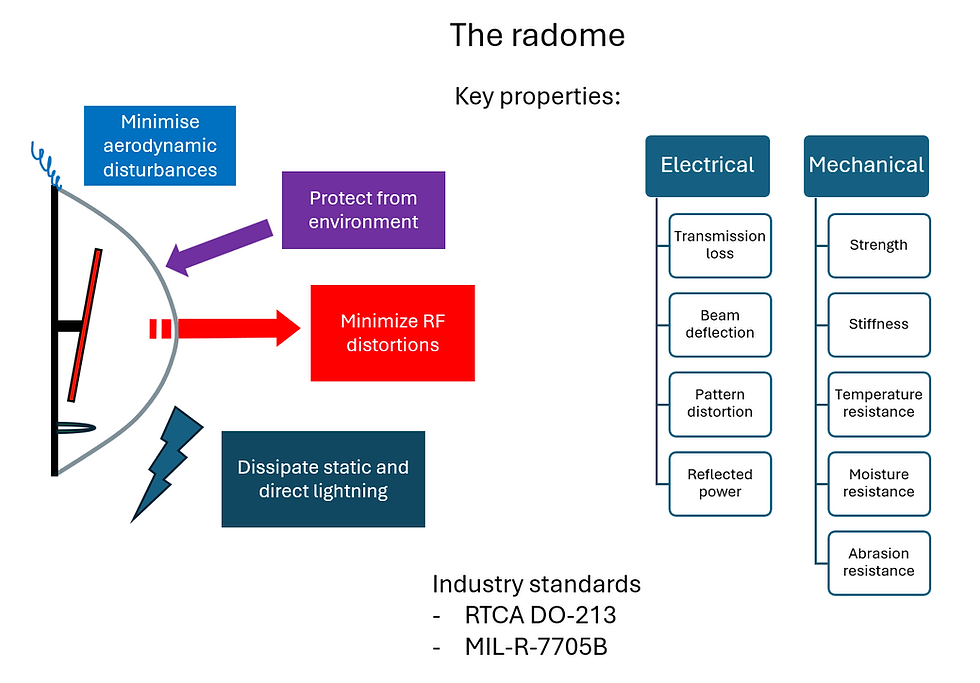
Radome materials
Radomes are typically made of fiberglass or Kevlar; the resins include polyester and epoxies [1]. The thickness and wall construction are critical as they greatly affect the RF characteristics. It is not surprising that a radome is specifically designed for a particular aircraft / RF-system combination. The main types of radome constructions are [2]:
Thin membrane, typically 1 mm or less. Panel flanges carry the structural loads
Solid laminate, around 2 mm thickness
2-layer sandwich, foam between thin membranes (typical for turboprops)
3-layer sandwich, composite foam core with a thickness of ¼ lambda of the highest RF frequency used
The coating is anti-static and hydrophobic. This is important to avoid static discharges creating pin holes and to prevent water from accumulating [2]. The effects of poor coating are especially visible in rainy conditions when water is able to form a layer on the radome surface. Many radome designs incorporate “diverter strips” (see Figure 1) for lightning protection.
RF-transmission efficiency
Oddly enough, there was no Minimum Operational Performance Standard (MOPS) for radomes in the civil world during the early days. The problem of differences in RF transmission efficiency manifested itself when the weather radars became more sophisticated and began to include predictive windshear functions [3]. A special committee (SC-173) was formed at the RTCA to develop such a standard, resulting in RTCA DO-213 [3]. Amongst other things, this standard stipulates minimum values for transmission efficiency [3]:

Historically, a typical issue was the retrofit of a predictive windshear radar into a legacy aircraft equipped with a low performance radome. This led to suboptimal performance of the radar [4].
Hidden damage?
Even small static burns or seemingly minor bird-strikes can have serious consequences on the integrity of the radome. The reason lies in the honeycomb-structure and the possibility of skin-delamination after repeated flight-cycles or moisture ingress. Aircraft manufacturers advise pilots to consult maintenance if such an event has occurred [3] [5].
Typical warning signs for pilots are:
Degradation of weather radar performance
Antenna drive unit failures which cannot be replicated on the ground
“Fake” weather returns
Cluttered radar display
Pilots should be vigilant for these symptoms, especially after a radome damage has been reported. A very good example can be found in [5]. Unfortunately, many of these symptoms cannot be replicated easily on the ground, making good communication between flight crews and maintenance providers an absolute necessity.
Testing after repair
RTCA DO-213 mandates electrical testing after repair, except if the radome damage does not exceed a specified size relative to the antenna aperture [3]. Apart from using the proper repair techniques it is vital to ensure testing is conducted, if required. Beware, that most maintenance facilities do not have such equipment readily available. Below is a sketch of a radome test setup:
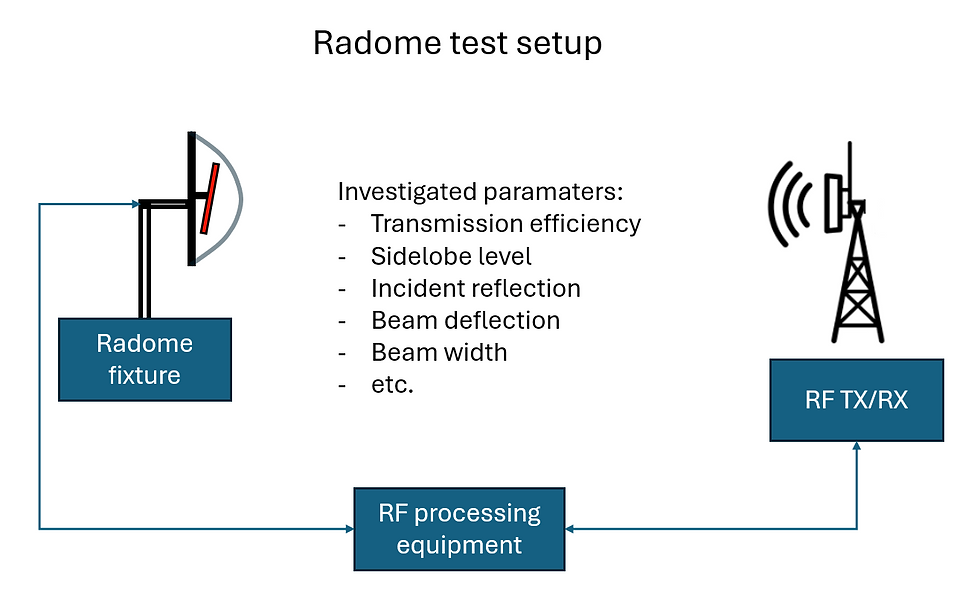
Typical in-flight consequences of radome damage
The effects of radome damage are various. They reach from structural damage, increased fuel consumption to unreliable air data. On Higher-Order-Flight-Control (HOFC) aircraft, the flight control law might also be degraded. The flight crew will be confronted with a challenging situation, seriously degrading the aircraft capabilities. The graphic below depicts a summary of potential consequences, it is not exhaustive:
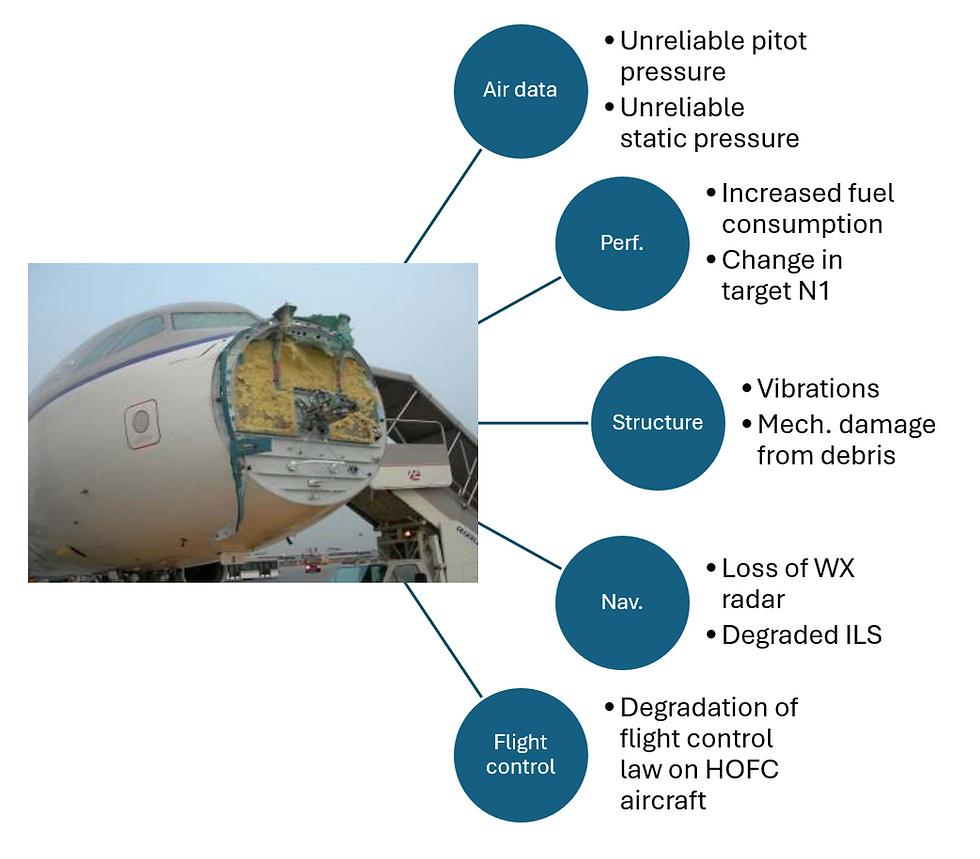
The situation is usually compounded by additional damage such as cracked windshields (if hail was encountered) which further complicate the situation. In case of limited forward visibility and if “autoland” is available, it should be considered. Depending on the extent of the damage this feature might not be available anymore…
Can we simulate it?
In today's world of sophisticated flight simulation and training devices (FSTD’s) one would think that radome damage can easily be simulated. Well, not quite. The main reason lies in the uniqueness of each event and the associated consequences. While the FSTD’s may be able to simulate a blocked pitot-tube, the air-data distortions after a radome damage are far more complex. As the examples below demonstrate, a damaged radome is usually accompanied by a plethora of cautions and warnings in a modern cockpit. So, while a simulator may be able to replicate some aspects of a radome damage, it will never exactly match the real event and flight crews will have to apply basic airmanship to solve the potentially confusing situation.
Incident example 1: Radome collapse, based on [6]
In 2010, a Fokker 100 aircraft was climbing out of Warsaw when suddenly, the flight crew heard a strange noise and observed unreliable air data and the following cautions:
Airspeed miscompare
No Autoland
Rudder limit
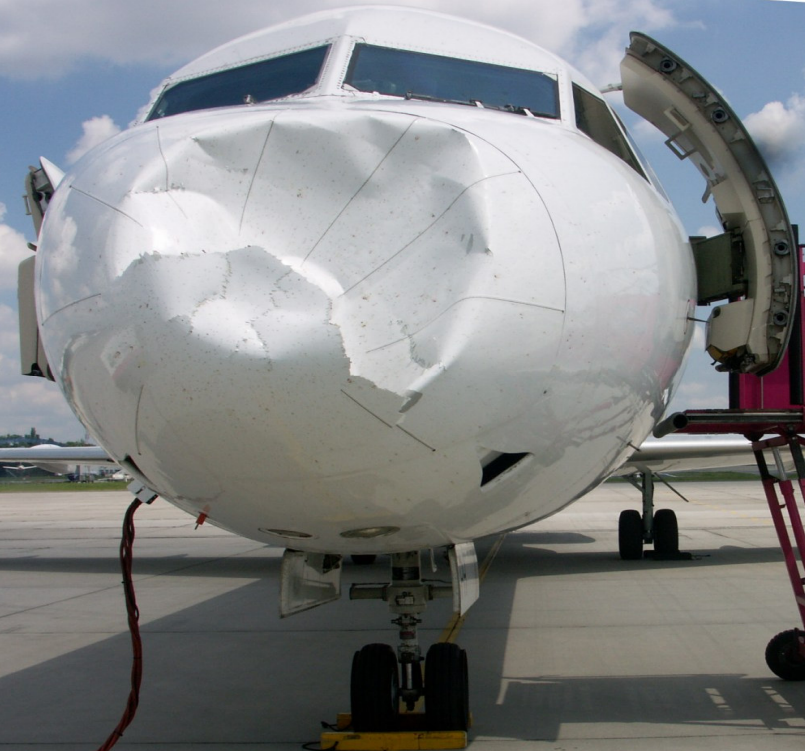
The flight crew decided to return to Warsaw for landing. Autopilot and autothrottle were used, but the autothrottle performed unsatisfactorily. During the final approach the windshear warning was triggered, and the flight crew conducted a go-around. Together with a duty officer who was standing near the landing runway, a radome damage was detected and the crew realised that the windshear warning had been erroneous. A second approach with successful landing followed. Interestingly: The investigation concluded that on the day of the event, there was no actual impact on the radome. The structure had been weakened by earlier undetected damage (probably bird strikes), allowing water to enter the honeycomb structure leading to structural degradation.
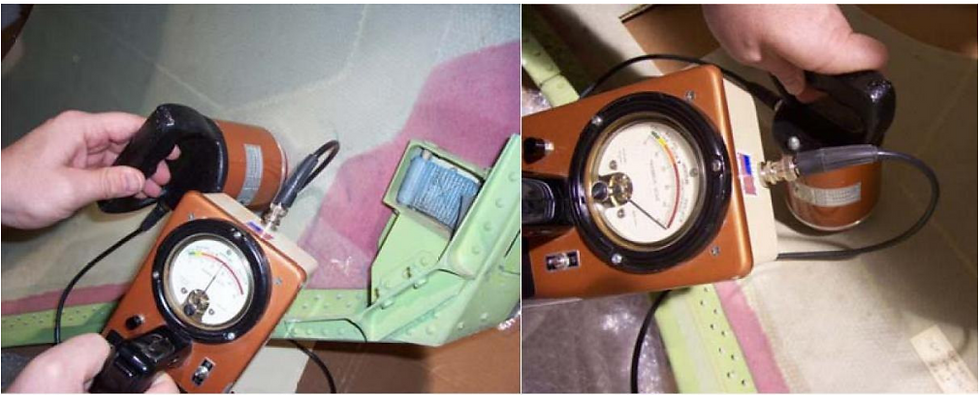
The investigation committee was able to confirm excessive moisture inside the radome as shown above. This gradually deteriorated the strength of the radome to the point where is simply collapsed during normal flight.
Incident example 2: Hail encounter, based on [7]
In 2006, an Airbus A321 was descending for approach at Gimpo airport Korea in convective weather. The aircraft entered a hail shower at an altitude of about 11’500 ft with a speed of 325 KCAS (!). The windshields cracked, and several aircraft systems were immediately degraded. The radome detached completely:
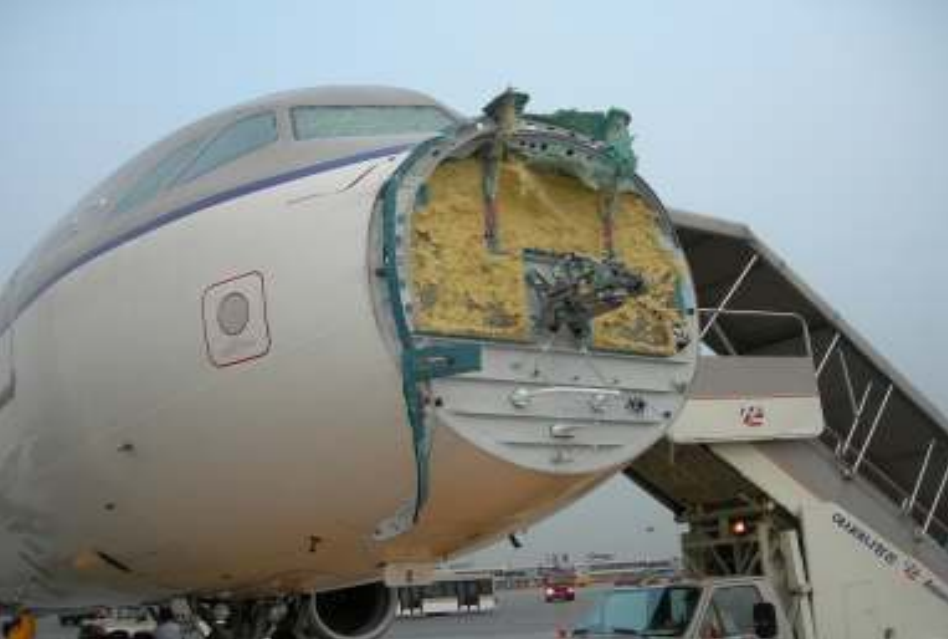
The crew were confronted with 34 Electronic Centralized Aircraft Monitor (ECAM) cautions/warnings. A selection is presented below:
AUTO FLT AP OFF
AUTO FLT A/THR OFF
NAV ADR disagree
ANTI-ICE L+R windshield
FCTL ALT LAW
AUTO FLT RUD TRIM 1+2 FAULT
During the encounter, the noise was so loud that a conversation was almost impossible. The captain’s airspeed showed 330 kts, while the F/O’s airspeed was 240 kts. The crew then attempted two hasty approaches to land which both had to be abandoned. Only on the third attempt a landing was possible with very little fuel remaining. Forward visibility was severely impaired as the pictures below show:
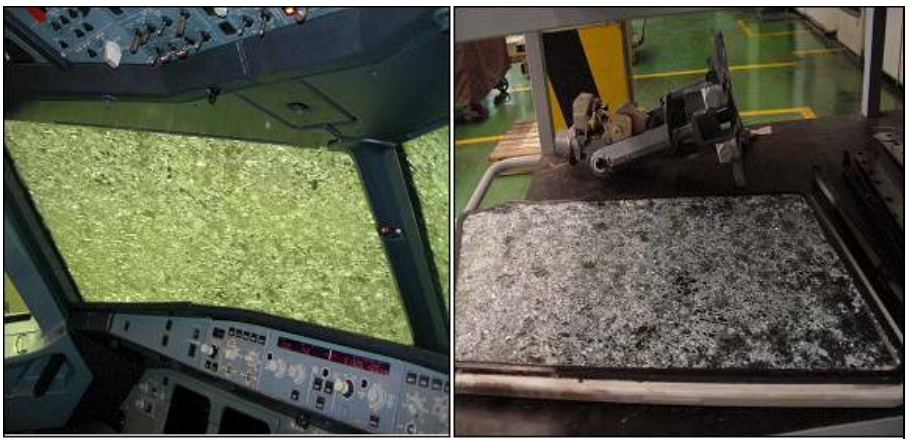
The incident highlights the importance of sufficiently avoiding convective weather and reducing airspeed appropriately. Should damage occur, sufficient time should be taken to analyse the situation and setting up for an approach in a coordinated manner.
References
[1] | P. Tusharkanti , S. Toofan und D. P. Smaraka Majhi, «Performance Study of various Aircraft Radome,» UGC Care Journal, Bd. 10, Nr. 2, pp. 174-178, 2020. |
[2] | V. M. e. a. Souma, «DESIGN CONSIDERATIONS OF RADOMES: A REVIEW,» International Journal of Mechanical Engineering and Technology, Bd. 8, Nr. 3, pp. 42-48, 2017. |
[3] | RTCA, «DO-213: Minimum operational performance standards for nose-mounted radomes,» Bd. 1, 1995. |
[4] | Aviationpros, «What you should know about radomes,» 1 02 1999. [Online]. Available: https://www.aviationpros.com/home/article/10389057/what-you-should-know-about-radomes. [Zugriff am 18 8 2024]. |
[5] | AIRBUS, «Bird or Hail Strikes on the Radome,» Safety first, April 2024. |
[6] | POLAND STATE COMMISSION ON AIRCRAFT ACCIDENT INVESTIGATION, «Serious Incident Occurrence No: 619/10 Aircraft: F-100, D-AGPH,» Warsaw, 2011. |
[7] | Korea Aviation and Railway Accident Investigation Board, «Aircraft Accident Report Hail Encounter During Approach Asiana Airlines Flight 8942,» Korea Ministry of Construction and Transportation , Seoul, 2008. |